1. Introduction. Predicting hot zones in catalytic reactors is the focal point of design criteria aimed to avoid severe safety hazards. Thermal fronts are known to exist in such reactors and some experiments indicate that they may undergo symmetry breaking in the transversal direction under certain conditions. Note, that the linear stability analysis of the 1-D front solution is a rather complex problem as the basic (steady or moving) front state is not known, a priori. Reported studies employ numerical simulations
1 which are very time consuming and are difficult to analyze. To simplify the full 3-D problem analysis several reduced models yielding a 2-D presentation have been proposed
2-3. Linear stability analysis can be applied then after a single, or multiple steady state (SS) solutions have been determined. The only analytical result obtained with such reduced models, in the case of a single stable SS is that the necessary condition for emergence of stationary transversal patterns is: Pe
Ctrans < Pe
Ttrans2. This is exactly a condition of the Turing activator-inhibitor mechanism and can be applied for a stationary front, but it is considered to be unrealistic for fixed beds. A new criterion of transversal symmetry breaking of moving fronts has been recently proposed by us
4. Using both a learning two-tube model exchanging heat and mass, and a thin cylindrical annular 2-D bed model we obtained for a first order activated reaction the critical condition to be: α=ΔT
adPe
T/ΔT
mPe
C>1, (1) where ΔT
ad and ΔT
m are the adiabatic and the maximal temperature rises. This condition coincides with the previous condition for stationary fronts (ΔT
ad=ΔT
m) when ratios of transversal to axial dispersions of heat and mass are consistent (Pe
Ctrans/Pe
C=Pe
Ttrans/Pe
T). The novel condition (1) can be satisfied in an upstream propagating front (ΔT
ad/ΔT
m>1) for feasible operating conditions (Pe
C>Pe
T) as was verified by direct numerical simulations of a 2-D shell PBR model
4. To implement condition (1) we need to know relation ΔT
m=ΔT
m(ΔT
ad,Pe
C,Pe
T), which to the best of our knowledge is not available yet. The purpose of this study is to derive the approximate relations for the propagation velocity and the maximal temperature rise of a planar front in a PBR, accounting for a finite mass dispersion and to analyze transversal symmetry breaking of moving and stationary fronts using the relation between the velocity (Vf) and the local curvature (K) of the front. 2. Analysis. As a first stage we derived for a planar front two algebraic equations with two unknowns (ΔT
m,Vf), which in the limiting case of Pe
C→∞ can be reduced to the approximate relations known for a 1-D "ideal" front in the case of negligible mass dispersion
5. Numerical simulations of a 1-D PBR show a good agreement between the simulated and approximated values for moderate and large Pe
C. At the next stage we considered a curvilinear front propagation in a local polar coordinate system and derived approximate relations for the propagation velocity (Vf
c) and the maximal temperature rise for the case of a finite curvature. Applying the condition dVf
c/dK|
K=0=0 we obtained a necessary condition for symmetry breaking in the following form: C(α,ΔT
ad,Da,Pe
T,Pe
C,ΔT
mc,Vf
c,γ)[1-α] <0, (2) where C is a certain algebraic expression. In the limiting case α→0 (Pe
C→∞) we have C>0 and the planar 1-D front is stable. Analysis of simplified Eq. (2) with α>1-δ (δ>0, small) shows that C>0 at least for 1< α <2.4 and thus a planar front is unstable within this domain. Note, that condition α>1 coincides with the previously obtained criterion (1), while refined condition (2) can define the upper α boundary of transversal patterns to emerge. 3. Simulations. The derived criterion was verified by direct numerical simulations of the 2-D cylindrical shell and a full 3-D PBR models. With both models simulations revealed formation of transversal patterns in upstream propagating fronts. On the cylindrical shell the sustained patterns include "frozen" multi-wave, rotating and aperiodic structures. In the 3-D PBR model rotating spirals are generic forms of transversal patterns. The wave structure becomes more complex with increasing the ratio Pe
trans/Pe. In conclusion we would like to point that transversal three-dimensional patterns were predicted and simulated in PBR's with a first order activated kinetics within the feasible domain of parameters for the first time. Figure 1.Typical front transformation on an unfolded cylindrical shell leading to formation of upstream propagating transversal patterns (moving coordinates). Figure 2.Typical rotating spiral structure of a front propagating in a 3-D PBR showing the temperature pattern in a cross-section normal to the flow (behind the front). References 1. V.Balakotaiah, E.L.Christaforatou, & D.H.West, Chem. Eng. Sci., 54, 1725-1734 (1999). 2. G.Viswanathan, A. Bindal, J. Khinast, & D. Luss, D, AIChE J., 51, 3028-3038 (2005). 3. V.Balakotaiah, N. Gupta, & D.H.West, Chem. Eng. Sci., 57, 435-448 (2002). 4. O. Nekhamkina, & M. Sheintuch, Chem. Eng. Sci, in press. 5. O.V. Kiselev, & Yu. Sh. Matros, Combustion, Explos. and Shock Waves, 16, 152 (1980).
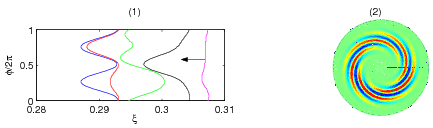