Methanol, lower hydrocarbons, CO, and HCOOH formation have been by the reduction of CO2 with H2. Un-catalyzed electro-reduction requires a significant overvoltage. An alternate route has been conceptualized. Production of hydrogen from water can be achieved by chemical oxidation of a metal in water followed by oxidation of the hydronium ion by the released electrons to form hydrogen radical. It is hypothesized that circumventing the problem of the formation of hydrogen molecule is essential while having the hydrogen radical to react with CO2 forming methanol, etc. This may be possible by a bimetallic catalyst acting as a galvanic couple, wherein one metal acts as the electron donor for the production of hydrogen radical while the other acts as a catalyst for the reduction of CO2. This may enhance the reaction rates. The current concept circumvents the need for an external electric field unlike the electrochemical process. Utilization of carbon dioxide as a raw material, not only as carbon sources but also as useful chemical feedstock is a more holistic approach to reduce air pollution by flue gases. Several methods have been proposed to maintain the concentration of atmospheric CO2 or to reduce and recycle it. One of these is the chemical fixations using catalysts. The conversion of CO2 to methanol by hydrogenation over copper containing catalysts is one of the most widely studied subjects in this area. The limitation in this reaction is the low conversion of CO2. Methanol is currently produced from CO-rich synthesis gas (CO/CO2/H2) using a catalyst at 50¨C100 bar and 473¨C523 K. CO2 has been identified as a carbon source for methanol synthesis based on the fact that kinetic experiments, using isotope-labeled carbon oxides and spectroscopic methods, have demonstrated that methanol is produced from hydrogenation of CO2, whereby CO merely provides a source of CO2 and acts as a reducing agent by scavenging surface oxygen. Nevertheless, the catalyst that is active for CO-rich feedstock is not so active for CO2-rich sources. There have been many claims that the catalyst performance can be improved. The majority of the modified catalysts still contain Cu and Zn as the main components, together with different modifiers such as chromium, zirconium, vanadium, cerium, titanium, and gallium. The use of these catalysts leads to a more reduced state for the catalyst surface, a situation that may further facilitate surface hydrogenation reactions. Methanol synthesis from a CO2/H2 feed has already been achieved by either physically mixing a CuO-ZnO catalyst with a Pd catalyst or by using Pd-impregnated CuO-ZnO-Al2O3 catalysts instead of physical mixtures. In spite of the advances in direct hydrogenation technologies, the commercial implementation of these technologies is greatly hindered due to the cost prohibitive state of art of hydrogen production. In plants generating hydrogen as a fuel source, the utilization of this hydrogen in reducing CO2 is counter productive. Thus alternate methods need to be investigated that can convert CO2 to useful chemicals without the use of molecular hydrogen as the reducing agent. Two such processes currently under investigation are the electrochemical route of reducing CO2 at the cathode surface and the photo catalytic route. Both these routes utilize water as the source of hydrogen (that is generated in situ). Another method that utilizes water as the source of hydrogen to reduce CO2 is the use of zero valent iron. However, it was reported from the studies with zero valent iron that methane was the primary product. Direct methanol production from CO2 may be commercially feasible with an inexpensive source of H2. As mentioned earlier, use of hydrogen for this reduction chemistry is neither economically attractive nor is it the preferred route when the primary product is hydrogen. Hydrogen may be replaced by electrons and protons, available, for example, in electrochemical reduction in aqueous media. One-electron reduction of carbon dioxide to the radical anion CO, presents thermodynamic and kinetic barriers. In aqueous solutions the reduction potential is -1.9 V vs. SHE. The barrier to outer-sphere electron transfer for the couple is large because of the very different geometries of the linear, neutral carbon dioxide and the bent radical anion. Thus, direct (un-catalyzed) electro-reduction requires a significant overvoltage. This thermodynamic barrier can, however, be reduced by protonating the reduction product. [CO2 + 6H+ + 6e = CH3OH + H2O (-0.38 V)] An alternate route has been conceptualized. The production of hydrogen from water is achieved by chemical oxidation of a suitable metal in the presence of water followed by oxidation of the hydronium ion by the released electrons to form hydrogen radical. In the presence of a suitable catalyst, it may be very efficient in reducing carbon dioxide to methanol. If such a catalyst is not present in the vicinity of its production, it may combine with another hydrogen radical to form hydrogen molecule. To circumvent the problem of the formation of hydrogen molecule (which is less reactive than the radical), it is hypothesized that a bimetallic catalyst, wherein one metal acts as the electron donor for the production of hydrogen radical while the other acts as a catalyst for the reduction of CO2, may be utilized. If the bimetallic catalysts acted as galvanic couples such that the hydrogen radical was being produced on the catalysts while the other metal participated in maintaining the oxidation state of the catalyst as zero, then the reaction rates are further enhanced. The process is based on the ability of certain transition metals (M1) to oxidize in the presence of water giving of electrons that in turn converts the hydronium ion into hydrogen. This hydrogen is then adsorbed locally onto another metal (M2) of known catalytic activity towards CO2 hydrogenation. This process would follow the same route as that of an electrochemical route for CO2 hydrogenation with the added advantage of circumventing the need for an external electric field. Table 1 shows the mechanism for CO2 conversion to methanol. In this scheme, it is possible to have competing reactions that produce intermediates such as formats and bicarbonates as well as hydrocarbons as demonstrated by researchers working with a single metal catalyst. However, these intermediates would also be reduced by the highly active hydrogen radical/metal hydride formed in situ and it is expected that hydrocarbon formation may be reduced/eliminated with proper design of the catalyst and the process. It must be noted here that the catalyst M2 may also be oxidize in a similar fashion as M1 depending on the choice of the catalyst. In this case the hydrogen radical would be formed on the M2 part of the bimetallic catalyst. If M1 is chosen such that it can form a galvanic couple with M2 , the reaction rates would further be enhanced. The selectivity of different galvanic products towards various end products will be presented. The presentation will also illustrate the mechanism of methanol production from carbon dioxide using galvanic couples. The thermodynamic approach adopted to identify suitable electron donors and catalysts will also be presented and the galvanic couples will be identified. The experimental procedure to synthesize the nanoscale galvanic couples will be shown along with the characterization data of the produced nanosized catalysts. We have found that the use of such bimetallic galvanic couples enable the reactions to process at a much faster rate than the corresponding electrochemical routes. In addition, synergistic activity was observed when the catalyst form a galvanic couple as opposed to the performance of the catalysts individually. Depending on the galvanic couple used, the end product may be methane or FT hydrocarbons or methanol. Results pertaining to the performance of the bimetallic catalysts in terms of their reactivity towards CO2 hydrogenation and their selectivity for methanol synthesis will be presented along with the future experimental plan to understand the mechanism of the reactions and activity of the catalysts. Both aqueous media and gas phase reactions are being considered. In the presented results, the effects of process parameters such as temperature, pressure, gas flow rates, pH (in aqueous media) will be shown. In addition, the effect of impurities present in the CO2 stream will also be shown. Table 1 Mechanism of CO2 Conversion to Methanol over Bimetallic Catalysts
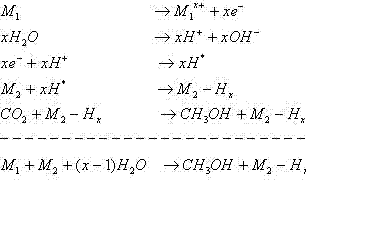